Simulations reveal non-volatile means of topological order control in 2D heterostructures
by Carey Sargent, EPFL, NCCR MARVEL
Quantum spin Hall insulators (QSHIs) are a class of 2D topological insulators that dissipate very little energy while allowing electrons to flow along the edge. This makes them ideal materials for development into ultra-low power devices.
One of the unique characteristics of the material is that the electron spin, i.e., its intrinsic magnetic moment, depends on the direction the electron is moving. This makes QSHIs ideal for new, so-called spintronic devices. In such devices, the spin Hall effect converts the current formed by electron charge into current based on electron spin. The materials could therefore power various applications: they could host 1D low-dissipation wires for use in nanoelectronics such as interconnects and the spin-momentum locking of the edge states could also be exploited in spintronic devices such as spincurrent generators and charge-to-spin convertors.
Many potential applications—the topological field-effect transistor (topoFET) is one example—rely, however, on the possibility of switching between a topological and a trivial insulating phase through the application of an electric field. Generally, the system is a QSHI when no electric field is applied and edge conductance is turned off for a sufficiently strong gate voltage, but the opposite can also be true. In either case, the system generally goes back to the zero-field state when the gate voltage is removed—energy consumption must be maintained. Developing an approach that allows the material to stay in the topological or trivial state even after the field is removed would be a huge boon to the development of such devices.
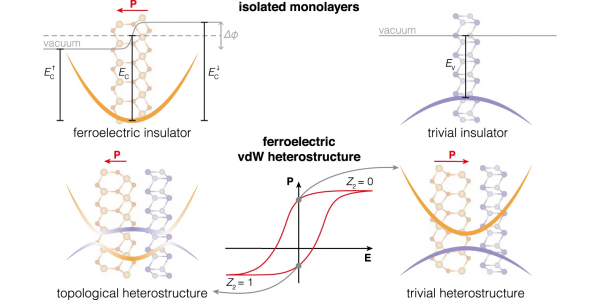
One way of introducing this memory would be to incorporate ferroelectricity. This is a characteristic of certain materials having a spontaneous electric polarization that can be reversed by the application of an external electric field. Ferroelectric topological transistors would consume energy only to switch states and would preserve the memory of the state, allowing for low dissipation storage devices, for instance.
The coexistence of ferroelectricity and topological order is rare, however. On top of this, in bulk ferroelectric materials, the two polarization states are related by inversion symmetry and so the topological order is identical in both states. In antiferroelectric topological insulators, topological transitions require a finite field to be sustained and would exhibit the same topological or trivial phase for opposite field directions. For the applications at hand, it would then be better to have a ferroelectric structure where opposite polarization states (at zero field) correspond to different topological phases, enabling the non-volatile control of edge currents. Where to find this though?
In the paper “Twist-resilient and robust ferroelectric quantum spin Hall insulators driven by van der Waals interactions,” recently published in npj 2D Materials and Applications, researchers Dr. Antimo Marrazzo and Dr. Marco Gibertini, both formerly of NCCR MARVEL, and now in Italy at the Universities of Trieste and Modena, respectively, propose combining a ferroelectric layer with another two-dimensional (2D) trivial insulator.
Their idea was inspired by In2ZnS4, a material that the authors recently identified as a QSHI. The structure of this material can be interpreted as being made of two semiconducting and topologically trivial monolayers, In2S3 and ZnS. In2S3 is polar, however, and the band offset associated with the vertical electric dipole leads to an inversion between the valence band that’s associated with one layer, and the conduction band, associated with the other, and the two hybridize with the appearance of a topological gap in the presence of spin-orbit coupling (SOC).
The researchers started from the premise that if the polar material is ferroelectric, a material made of two topologically-trivial 2D materials–namely a trivial insulator and a ferroelectric insulator–will behave as a ferroelectric QSHI where the polarization direction and the Z2 topological invariant are coupled. This arises from the fact that the valence and conduction bands are associated with different layers and that the two polarization states, with opposite offsets stemming from the vertical dipole, give rise to different alignments between them.
Though the idea was initially inspired by In2ZnS4, the material showed poor performance in terms of band gap. The researchers rather chose the well-known ferroelectric semiconductor In2Se3, as a basis. They then needed to combine it with an optimal semiconducting monolayer from large databases of 2D materials, focusing on easily exfoliable compounds. To make simulations easier, they looked for a material lattice matched with In2Se3; in which the top of the valence band is sufficiently close in energy to the conduction band bottom of the other material and which contains sufficiently heavy elements to be likely to display significant SOC. While these conditions might seem very strict, the authors say, there are actually several satisfactory candidates according to certain density-functional theory (DFT) simulations. In the end, they chose to form the heterostructure with an easily exfoliable trivial insulator, CuI.
Using first-principles simulations, they went on to show that the approach could indeed be used to engineer non-volatile ferroelectric control of topological order in 2D heterostructures. The effect is robust and can survive up to room temperature.
They also found that when the band inversion occurs at the Brillouin zone (BZ) center, its existence and the associated topological phase are purely driven by band alignment, and so are independent of the relative orientation of the two layers and don’t require lattice matching. This suggests, the researchers say, that while stringent conditions on band alignment and sufficiently strong SOC in at least one of the two materials are required, the range of possible material combinations is rather large.
“We stress that this combination of materials is chosen here only for illustrative purposes and that the physics we discuss is very general and it holds for a number of other systems,” the authors said. “We thus believe that there is an entire portfolio of prospective heterostructures to be considered in experimental investigations.”
Reference:
Marrazzo, A., Gibertini, M. Twist-resilient and robust ferroelectric quantum spin Hall insulators driven by van der Waals interactions. npj 2D Materials and Applications 6, 30 (2022).
https://doi.org/10.1038/s41699-022-00305-9
Low-volume newsletters, targeted to the scientific and industrial communities.
Subscribe to our newsletter