The search for topological materials in MARVEL: a joint effort of theory and experiment
by Nicola Nosengo, NCCR MARVEL
The 2010s were a golden age for research on topological materials, one of the most interesting and elusive manifestations of quantum properties in materials at the macroscopic scale. Topological materials are unusual phases of matter that owe their behaviour to the geometrical properties of their electronic structure, in particular the immunity of charge carriers to various types of disturbances. Many hope that topological materials could be used in new generations of electronic devices, or in future quantum computers, and their discovery was behind the 2016 Physics Nobel Prize to David J. Thouless, F. Duncan M. Haldane and J. Michael Kosterlitz.
It is not surprising then that NCCR MARVEL paid great attention to this line of research since its start in 2014, and then dedicated a whole “design and discovery” project to topological materials during the second phase.
“When we started there were very few known topological materials” recalls Oleg Yazyev, Associate Professor of Computational Condensed Matter Physics at EPFL who, as a MARVEL member, led this line of research from the theoretical side. “A few had been predicted theoretically and then confirmed experimentally, and that drove people like me to the field. We expected to find some new materials very quickly and advance them to technological applications. We largely succeeded in attaining the first half of this objective but the second turned out to be not that simple”.
The initial step, led by Yazyev, was a high-throughput computational search for topological materials based on a screening of over tens of thousands compounds in the International Crystal Structure Database (ICSD). The effort resulted in a database of topological materials that became part of the Materials Cloud and that is still in high demand. “There are other similar databases around. We are not alone, but we were among the very first. We are glad to see that the results of our efforts are open to everyone, and continue to be used by many groups worldwide”.
The high-throughput search pointed towards a few particularly promising candidates, at which point theorists joined forces with experimentalists to investigate them and confirm their topological properties.
“Our first success was the discovery of bismuth iodide as a topological insulator, something we had first predicted theoretically and then confirmed experimentally” recalls Yazyev. “Instead of immediately publishing the prediction, we chose to assemble a team of experimentalists and we managed to pull out a publication where theory, synthesis and characterisation appeared together”.
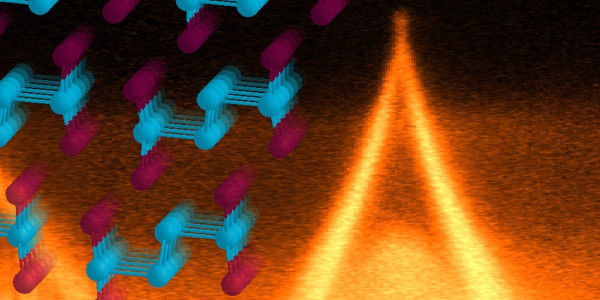
Topological surface states in bismuth iodide © O. Yazyev (EPFL)
Around the same time, to strengthen the experimental expertise on novel materials, the laboratory of Ming Shi at the Paul Scherrer Institute joined MARVEL. “By that point the insulator phases had been thoroughly studied, and there were two main directions to go” says Ming Shi, who has since moved to Zhejiang University in Hangzhou, China. “One was to study correlated systems, where you don’t only consider the electrons in isolation. The other one were Weyl semimetals”.
Weyl semimetals are topological materials with unique transport, optical and thermoelectric behavior, due to the fact that their electrons behave as if they are massless due to the presence of nodes in the electronic band structure.
The first result of the collaboration between Yazyev and Shi was a combined theoretical and experimental study comparing the Weyl nodes in tantalum phosphide (TaP) and in tantalum arsenide (TaAs), another topological system that had been characterized previously. The different patterns found in the two semimetals, and the fact that in TaP only one type of Weyl nodes contribute to the anomalous low-energy physics (as opposed to two types in TaAs) was an important contribution to the understanding of the physics of Weyl semimetals.
Then came the major result with the collaborative study of tungsten phosphide (WP2), which was shown to be a type-II Weyl semimetal – a system, first described by Alexey Soulyanov, where the Weyl nodes appear at the touching points of electron and hole pockets. “The material had been predicted by Oleg’s group and we did the verification, it was very nice collaborative work” says Shi. The paper ended up in Physical Review Letters, highlighted by the editor.
The study combined first-principles calculations and soft X-ray angle-resolved photoemission spectroscopy (SX-ARPES) and found, as predicted by density functional theory (DFT) calculations, that the material is a robust type-II Weyl semi-metal.
“The electronic structure calculations that allowed us to uncover new topological materials were quite basic, pretty standard DFT”, says Yazyev. “More sophisticated techniques such as GW were only used to scrutinize what we call ‘candidate’ materials. The innovation was identifying the right topological indices, especially for tricky materials”.
As for the experimentalists’ toolbox, Ming Shi explains that “when it comes to confirming theoretical predictions on 3D materials you always need the electronic structure, and ARPES is the most direct way to obtain it. At the time it was a rather difficult technique, though nowadays it has become easier”. One problem, he explains, is the quality of the sample: in the early phases of experiments on a new material one cannot know if the samples you’re working with are good or bad. Another big difficulty in applying this technique to Weyl semimetals was to find out the surface states of the material. “You need to find the Fermi arcs, which means a Fermi surface that is only partially closed”, he says. “That requires some judgement on the experimentalist’s part. But now this technique is quite well-documented and things have become easier”.
Ming Shi is still researching topological materials, focussing on more challenging problems such as highly correlated systems, frustrated systems, or highly exotic topological phases such as quantum spin liquids.
As for Yazyev, after leaving MARVEL at the end of Phase 2, his work has gradually shifted to other areas of research and activity on topological materials in his group has decreased. Part of the reason is that topological materials such as bismuth iodide or tungsten phosphide have not yet found technological applications, and enthusiasm for the field has cooled down.
“Unfortunately materials do not play out like idealized models” he says. “Crystalline materials have plenty of complications, like intrinsic defects, and at the moment it’s difficult to find materials that behave nicely enough”.
Still, says Yazyev, the insight gained from the work on topological materials has spilled out on other systems, such as photonics, microwave circuits, topoelectrical circuits and other realizations of “synthetic” matter. And the fact that no commercial application of topological material has been found yet does not mean that it can’t happen in the future, and that enthusiasm for the field can’t grow once again. “These things come in waves, like it happened for graphene”, says Yazyev. “What matters is that we’ve made our contribution to the field and we can be proud of it”.
Low-volume newsletters, targeted to the scientific and industrial communities.
Subscribe to our newsletter