A paradigm shift in calculating the spectral properties of semiconductors
By Nicola Nosengo - NCCR MARVEL
Materials scientists have made enormous progresses in the last decades in their capacity to simulate, explain and predict the properties of materials using computational tools that rely on the fundamental laws of quantum mechanics. But there are still blind spots, and one of them has to do with calculating the spectral properties of some semiconductors.
The staple method for materials simulation, density functional theory (DFT) can effectively predict the properties of many materials at their ground state, which is the stable state at the lowest possible energy level. But it does not give access to spectral properties, such as how the electrons of a material interact with light (i.e., photons). There are other theories that can solve the problem, like Green’s functions and many-body perturbation theory, often based on the so-called Feynman diagrams. But they are very complex and often do not treat consistently spin, that is a fundamental property of particles and atoms, especially relevant for magnetic behavior.
“Dealing with spin degrees of freedom is difficult on a theoretical level, and computationally very expensive, especially within advanced electronic structure approaches” says Nicola Colonna, a scientist in Nicola Marzari’s NCCR MARVEL laboratory at the Paul Scherrer Institute in Villigen, Switzerland. The limitations of current methods become particularly relevant for materials, such as semiconductors, that have a strong spin-orbit coupling, a relativistic effect that is particular evident in heavy chemical elements that often play a major role in cutting-edge scientific or technological applications.
In a new article just published in Physical Review Research, Colonna and Antimo Marrazzo from Scuola Internazionale Superiore di Studi Avanzati (International School of Advanced Studies) in Trieste, Italy, advocate for a paradigm shift by introducing a new approach that allows calculating band structures of semiconductors in a simple way and at low computational cost, even in presence of spin-orbit coupling or complex magnetic configurations. The band structure is a fundamental quantity that is key to describe many properties of semiconductors, ranging from light absorption to electronic transport, and is the main workhorse of semiconductors physics.
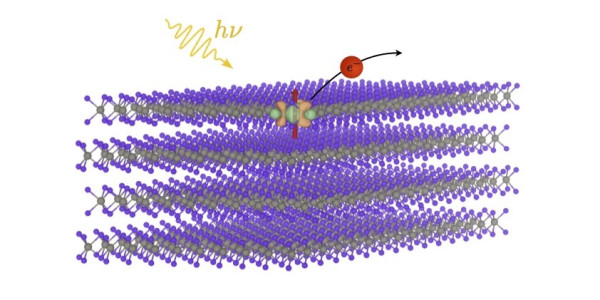
The starting point is a method pioneered by Marzari’s group to include spectral calculations into DFT, called Koopmans functionals. The key idea of Koopmans functional is to move from a functional theory of the total electronic density (i.e., DFT) to a functional theory of individual orbitals densities, such that the energy that comes out of the theory should increase or decrease linearly when adding or removing an electron from the system. This Koopmans condition is naturally akin to that of enforcing a correct description of charged excitations and photoemission processes. So far the theory has not been capable to deal with materials with strong spin-orbit coupling, and the two authors expanded it to this specific case. “While DFT and Koopmans are based respectively on the total density of electrons and on the orbital density of each electron, here we added also the density of spins, or the density of magnetization” says Colonna. The result is a calculation that depends on significantly less variables than Green’s functions, and thus more manageable.
“When we started the project, our aim was to be able to simulate band structures (and spectral properties in general) in materials where relativistic effects are strong, so to get there we set sail for developing a dedicated orbital-dependent theory and implement the method in Quantum ESPRESSO” says Antimo Marrazzo, first author of the work. “But in the process, we actually ended up discovering and understanding a more fundamental and general aspect of the electronic structure: including spin in the effective interaction between electrons in a material is essential to describe accurately its spectral properties even in systems where spin-orbit coupling is weak or there is no magnetism at all, such as in silicon”.
“Our approach”, continues Marrazzo, “crucially include spin-dependent interactions and screening effects that are either missing or rather complex to include in competing approaches, while they emerge very naturally in our non-collinear orbital-dependent functional framework”.
The new computational method that resulted from this approach was validated on some well-studied materials such as gallium arsenide, a semiconductor with many known applications, and other systems characterized more recently such as CsPbBrl, a member of the perovskite family of materials that are excellent candidates for solar cells applications; tungsten diselenite (WSe2), that is interesting for his strong spin-orbit coupling and also because a 2D material can be obtained from it; and a material with strong magnetism in addition to spin-orbit coupling, CrI3.
The results of the calculations proved in very good agreements with other well-established but more costly and unwieldy theories, such as many-body perturbation theory, and with experiments.
The method has been developed and made publicly available in Quantum ESPRESSO, an integrated suite of open-source computer codes for electronic-structure calculations and materials modeling at the nanoscale. Quantum ESPRESSO is an open initiative, in collaboration with many groups world-wide, coordinated by the Quantum ESPRESSO Foundation that includes the Scuola Internazionale Superiore di Studi Avanzati (SISSA), the Abdus Salam International Centre for Theoretical Physics (ICTP), the CINECA Italian National Supercomputing Center, the Ecole Polytechnique Fédérale de Lausanne (EPFL), the Oden Institute for Computational Engineering and Sciences, The University of Texas at Austin, in partnership with the Italian National Research Council (CNR).
Colonna and Marrazzo say that this development will allow efficient and accurate computational screenings of materials databases and enable simulating complex materials under more realistic conditions, such as in presence of defects or at finite temperature. For future development, the group aims to apply the method to other quantum materials and keep exploring magnetic systems.
“We will teach our method to other scientists with dedicated workshops and summer schools” they say, “hopefully empowering them with a powerful new asset to understand, discover and design materials”.
Reference
Antimo Marrazzo, Nicola Colonna, Spin-dependent interactions in orbital-density-dependent functionals: Noncollinear Koopmans spectral functionals, Physical Review Research 6, 033085 (2024). https://doi.org/10.1103/PhysRevResearch.6.033085
Low-volume newsletters, targeted to the scientific and industrial communities.
Subscribe to our newsletter