ARPES gives first observation of dispersive excitons in a low-dimensional metallic system
by Carey Sargent, EPFL, NCCR MARVEL
Excitons, a bound state of an electron and an electron hole, can form when a given material absorbs a photon above its bandgap energy. The photon excites an electron from the valence band into the conduction band, leaving behind a hole. This positively charged hole attracts the electron and the two can become bound by an electrostatic force known as the Coulomb effect—this results in an exciton.
While the state itself is electrically neutral, excitons contain energy, meaning that they’re able to transport energy, but not net electric charge, through materials. This feature is already exploited in certain so-called excitonic solar cells: the cell absorbs light, creating excitons, which are diffused to an engineered material that can split them back into electrons and holes, releasing electrical energy. Understanding how and under what circumstances excitons move around—in this case, getting them to the engineered interface—is then critical in figuring out how to transport energy or information faster and more efficiently. More fundamentally, it’s also critical to our understanding of how light interacts with materials and of opto-electronic effects.
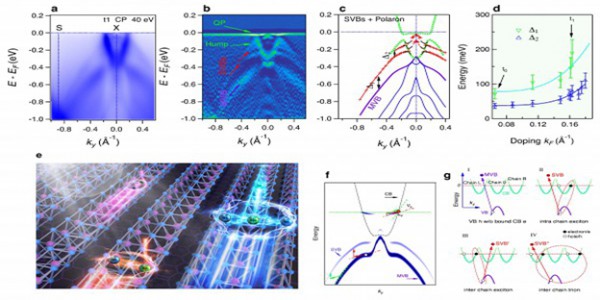
Figure 1: Multiple mobile excitons manifested as sidebands in quasi-one-dimensional metallic TaSe3. (a,b) The band structure determined from ARPES measurements and it’s curvature intensity plot along the X-S direction of 1D TaSe3. The data shows side valence bands (SVB) above the main valence band (MVB). (c) The band dispersion extracted from tracing the peak positions of the full spectrum in a and b. (d) The average energy separations between MVB and first SVB and between the first and second SVBs, plotted as a function of the Fermi wave vector, which is tuned by surface potassium doping. (e) Art sketch showing the bound states of intra-chain, inter-chain excitons, and inter-chain trions in 1D TaSe3. (f) The theoretical model, showing how the SVBs are constructed from bound states. (g) Proposed origin of the MVB and the various SVBs, with different inter- or intra-chain structures.
The trick, thus far, has been observing these movements. Traditional optical experiments have only created and detected excitons with negligible momentum. Using optical excitation to create non-moving excitons—that is, excitons formed by electrons and holes that located at the minimum and maximum of the conduction band and valence band, respectively—is standard in insulators. Researchers have even very recently detected dispersing excitons in the insulator monolayer WSe2. Observing mobile excitons with sharp dispersions in metals is another story though and detecting them has been difficult for many reasons: higher-dimensional metals are, for example, unlikely to even host excitons.
This led researchers led by NCCR MARVEL’s Ming Shi, professor and senior scientist at the Photon Science Division (PSD) of Paul Scherrer Institute (PSI), to focus their attention on low-dimensional systems in their quest to observe these mobile excitons. In the paper “Multiple mobile excitons manifested as sidebands in quasi-one-dimensional metallic TaSe3,“ recently published in Nature Materials, they specifically targeted the quasi-one-dimensional metallic trichalcogenide, TaSe3. The researchers deemed the Q1D material a prime candidate for probes of excitonic effects because of the interplay of dilute conduction electrons, low effective dimensionality and the presence of polarons, heavy quasiparticles whose mass increases the binding energy of the resulting excitons.
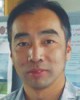
Using angle-resolved photoemission spectroscopy (ARPES), they systematically studied the metallic phase of the material. At low temperature, they found several side-valence bands (SVB) that appear exclusively above a pronounced valence band, with their dispersions roughly parallel to it. Their observations, the researchers say, suggest that the SVBs result from strong coupling between valence and conduction electrons and involve mobile bound states, that is, excitons, and maybe trions—mobile excitations that consist of three charged particles—that have not been observed so far using ARPES (although the trion induced valence band renormalization has been reported in semiconductors).
The excitons came with different internal structures, likely depending, the researchers said, on whether the involved holes and electrons belong to the same chain or to neighboring ones, or whether the hole binds one or two conduction electrons, resulting in an exciton or a trion, respectively. The interchain excitons are quasi-1D cousins of bilayer excitons in layered 2D materials, such as transition metal dichalcogenides, and are of particular interest because they may have a significantly longer lifetime than intra-chain excitons due to the spatial separation of the particle and the hole.
The scientists went on to investigate the effects of doping on the observed side-valence bands. Using potassium in situ on the cleaved surface of TaSe3 to increase the surface carrier density, they found that it raised both the binding energy and the typical momentum transfer in the conduction band. This increased, in turn, the energy separation between the SVBs and the MVB allowing, in effect, the tuning of certain exciton properties.
Reference:
Ma, J., Nie, S., Gui, X. et al. Multiple mobile excitons manifested as sidebands in quasi-one-dimensional metallic TaSe3. Nat. Mater. (2022).
Low-volume newsletters, targeted to the scientific and industrial communities.
Subscribe to our newsletter