New computational analysis introduces surface coverage as a descriptor for screening semiconductor catalysts for water splitting
by Carey Sargent, NCCR MARVEL, EPFL
Using a catalyst and sunlight to split water into its constituent parts has been proposed as an attractive means of generating the clean fuel hydrogen, which produces only water when consumed in a fuel cell. Though the scientific community has dedicated much time and effort to the topic, heterogeneous photocatalytic water splitting is still largely inefficient, making any large-scale commercial application impossible. The main stumbling block has been the lack of an efficient photocatalyst.
In principle, any semiconductor that is stable in an aqueous environment and able to harvest solar light could serve as a catalyst for the desired reaction. From this perspective, there are many suitable candidates. The possibilities start to narrow significantly however when adding the criteria characteristic of an ideal catalyst.
The best catalysts should have a favorable alignment of the band edges with respect to the redox levels of liquid water, a long lifetime and high mobility of the charge carriers. They should have high resistance to corrosion, a strong defect tolerance, and large ensuing photovoltage. Though it is not a criterion that has received much attention, they should also have a propensity to dissociate water molecules upon surface absorption. Spontaneous dissociation of water molecules in the form of ionic species like H+ and OH- in near-neutral conditions would be beneficial for promoting hydrogen or oxygen evolution and allow us to avoid extremely acidic or alkaline solutions, either of which could promote electrode degradation.
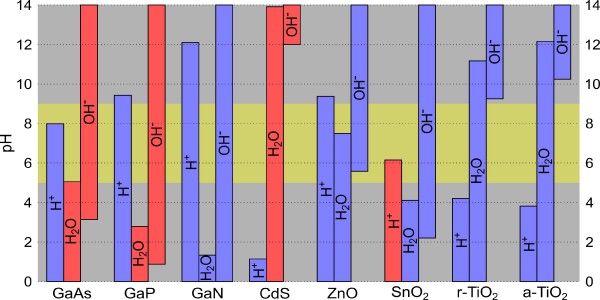
Figure 1:
Combined view on the surface coverage and alignment for the various semiconductors. For each adsorbate, the columns indicate the pH range where its surface coverage corresponds to a fraction of 10% or more, and the blue (red) color specifies a favorable (unfavorable) alignment between the band edges of semiconductor and the relevant redox levels. The horizontal yellow stripe emphasizes near-neutral conditions with 5 < pH < 9.
Practically speaking, however, it is impossible to test all of these properties for large numbers of candidate materials experimentally. Computational chemistry and physics have therefore been used to investigate some of the phenomena that are relevant to the efficiency of this process. This has allowed researchers to introduce some notion of design into the exploration of candidate materials. Advanced electronic-structure methods have facilitated the calculation of the band gap of semiconductors as well as the characterization of the alignment of band edges at the semiconductor-water interfaces.
Theoretical screenings of candidate materials have been used to define smaller sets of potentially promising photocatalysts with appropriate band gaps and band edges with respect to the redox levels of hydrogen reduction and water oxidation. Simplified interface models and, in some cases, more realistic semiconductor-water interfaces have allowed for the calculation of overpotentials associated with the water oxidation reaction. Still, describing the physical processes that occur at the semiconductor-water interface theoretically is complicated because they are multi-faceted—the processes depend on various factors such as the pH of the aqueous solution in which the material is immersed and defects at the surface, for example.
Simplified protocols that simply ignore such effects are likely to miss important physical aspects. This likely affects and could even invalidate the results. While it’s currently impossible to include all of the effects in a single simulation at the same time, recent theoretical approaches have at least been used to describing the acid-base chemistry and reactivity of pristine semiconductor-water interfaces. Identifying the characteristics of the ideal photocatalyst demands more extensive screening though—we need to model semiconductor-water interfaces at the atomistic level and consider criteria beyond band gaps and band edge positions.
In the paper “Evaluation of Photocatalysts for Water Splitting through Combined Analysis of Surface Coverage and Energy-Level Alignment,” the MARVEL team at the Chair of Atomic Scale Simulation including Zhendong Guo, Francesco Ambrosio, and Alfredo Pasquarello, used a combination of extensive ab initio molecular dynamics (MD) simulations and a formulation of adsorbates at the semiconductor-water interface to study acid-base chemistry and reactivity of eight different semiconductors at the interface with liquid water, for which interfacial structures and band alignments at their semiconductor-water interfaces had been determined previously.
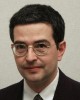
They used their calculations to determine the pH at the point of zero charge (pHPZC) and found excellent agreement with the results of experiments for all the semiconductors investigated. Calculating the acidic constant (pKa) of the individual adsorption sites at the interface allowed them to investigate the stability of adsorbed protons, hydroxyl ions, and water molecules at different values of pH for each material. Significantly, this computational surface coverage analysis provides a new descriptor that cannot currently be obtained by experiment.
In an additional step, the scientists aligned the band edges of the semiconductors at the interface with water. Combining this alignment of band edges with respect to the redox levels of the water splitting reaction with acid-base chemistry allowed them to examine the potential of each semiconductor to act as an efficient photocatalyst. It also allowed them to identify the optimal pH ranges of operation for hydrogen and oxygen evolution.
Among the eight materials considered, the approach identified gallium nitride, GaN, as the most promising photocatalyst for achieving overall water splitting in near-neutral conditions. The material could be made even more suitable through alloying, with, for example, zinc oxide (ZnO), the scientists said.
Reference:
Z. Guo, F. Ambrosio, A. Pasquarello, Evaluation of Photocatalysts for Water Splitting through Combined Analysis of Surface Coverage and Energy-Level Alignment, ACS Catalysis 10, 13186 (2020). [Open Access URL]
Low-volume newsletters, targeted to the scientific and industrial communities.
Subscribe to our newsletter